History of Planetary Development
(In addition to specific citations, see:
Douglas N. C. Lin,
"The Genesis of Planets," Scientific
American, May 2008: pages 50-59.)
Year 0 to 100,000 (0.1 Million) -
Solar
nebula collapses to form Sun
and
circum-Solar
disk
On October 4, 2006, a team of astronomers announced the finding
of evidence that Sol formed in a fragment
(Solar
nebula) of a
giant
molecular cloud (e.g., the
Orion Cloud)
of gas and dust that gave birth to a large
open star cluster
with hundreds to thousands of members. According to astronomer
Leslie Looney, the
evidence for Sol's stellar sisters was found in decayed particles
from radioactive isotopes of iron trapped in meteorites, which
can be studied as fossil traces of early Solar System conditions.
The isotopic evidence indicates that a supernova from a massive
star with the mass of at least 20 Solar-masses (probably a very
rare, hot, and blue
O-type
star like Anitak
Aa) exploded near the early Sun when it formed 4.6 billion years
ago. Measured abundances of the isotopic particle species indicate
that the supernova was located only about 0.32 to 5.22 light-years
from Sol. Where there are supernovae or any massive star, there
should have been hundreds to thousands of low-mass stars like
the Sun that were born of the same nebula of gas and dust. Due to
insufficient gravitational pull, Sol's surrounding cluster of stars
dispersed over the past five billion years as they moved around the
developing Milky Way galaxy, and members escaped the cluster due to
velocity changes from close encounters with each other, tidal forces
in the galactic gravitational field, and encounters with field stars
and interstellar clouds crossing
their way
(press
release).
John Bally,
Dave Devine, and
Ralph Sutherland,
STScI,
NASA
Larger false-color image.
Sol was probably born in a large
open star cluster near a massive
but rare, O-type star (like Theta1
Orionis C, the brightest of the
four central stars of the
Trapezium
Cluster in the Orion nebula, at
left) that exploded as a supernova.
On May 24, 2007, a team of astronomers announced that the
presence of an isotope of aluminium suggests the Sun was born when
an extremely massive star with around 30 Solar-masses released a
great amount of energy in winds loaded with
aluminium-26.
The strong winds of the massive star may have buffeted the Solar
nebula sufficiently to initiate the development of the Solar System
(Zeeya
Merali, New Scientist, May 24, 2007; and
Bizzarro et al,
2007; and
Shukolyukov
and Lugmair, 1993).
The high average abundance of gold in the Solar System suggests
that large amounts of the element was injected into matter that
eventually coalesced into the Solar nebula by the collision of
two neutron stars in a short-duration
gamma
ray burst (more from
Astronomy
Picture of the Day).
© Werner Benger,
Zuse
Institute Berlin,
Albert
Einstein Institute
(Artwork used with permission)
Larger illustration from a
movie.
The high abundance of gold in the
Solar System may have come mostly
from the collision of two neutron stars
in a close binary system (more from
Insights
Magazine and the
movie).
The Solar nebula of gas and dust became cold and dense
enough to collapse under the force of gravity and form our Sun,
Sol, by initiating nuclear fusion of core
hydrogen gas.
The developing Sun was surrounded by a
rotating
disk of leftover gas (mostly hydrogen and helium) and dust.
NASA
(Stapelfeldt
et al, 1999)
Herbig-Haro
object 30
(HH
30)
The Sun collapsed out
of the Solar Nebula
ringed with a disk of
infalling gas and dust,
much of which it spewed
back out in polar jets,
like those shown in this
video clip of young HH 30.
Year 0.1 to 1 Million -
planetesimals
form
Micron-sized dust grains or their more volatile
components (such as water) were vaporized in the hot and
denser region closer to the Sun, while those located in
the cooler and more tenuous outer region of the disk
grew from the condensation of vaporized heavier elements
around them. Particles located beyond the "snow line"
(between 2 and 4 AUs out from the Sun) grew faster
from the condensation of volatiles into icy grains,
while inner grains became rocky particles.
Dust particles are stirred by nearby gas and
collide with each other. Some particles grew and spiraled
inward, so that meter-sized bodies grew and moved halfway
towards the Sun within a thousand years.
Kilometer-sized bodies called
planetesimals
gathered up most of the original dust within one million
years.
Pat Rawlings, NASA
Larger image
Within the circum-Solar
disk of gas and dust, micron-sized
dust grains agglomerated into ever larger particles,
meter-sized boulders,
planetesimals,
then
planetary
embryos.
Some planetesimals survived and grew from gravity-assisted
collisions into ever larger
planetary
embryos until run out of
planetesimals within narrow orbital bands.
The size of planetary embryos grew with
distance from the Sun, due to the geometric growth
in the volume of surrounding planetesimals within
gravitational reach.
Evidence of an isotope of iron found in meteorites indicate that
a supernova exploded at around Year 1 million to inject
iron-60
into the young Solar System which later planetesimals and embryos
incorporated into their iron rich cores
(Zeeya
Merali, New Scientist, May 24, 2007; and
Bizzarro
et al, 2007).
According to astronomer Alan
P. Boss (Astronomy,
October 2006), many astronomers now believe that the development of
planetesimals into protoplanets as large as Earth's Moon (0.123
Earth-mass) was a runaway process, where a young Solar System may
have developed a swarm of hundreds of Lunar-mass protoplanets in as
little as 100,000 years. In a May 2008 update, astronomer
Douglas N. C. Lin
(Scientific American,
May 2008) notes that within around 1 AU from the Sun, embryos grew
to about 0.1 Earth-mass within 100,000 years.
Year 1 to 10 Million -
planetary
embryos form
At 5 AUs, embryos grew to around
4 Earth-masses within a few million years, but
embryos could grow larger near the snow line or
on the edges of gaps in the Sun's circum-Solar
disk where planetesimals also tend to accumulate.
NASA
Cassini-Huygens
Mission
to Saturn and Titan
Larger
image.
Jupiter
probably formed first
while the disk was still rich
in gas, acquiring half its mass
in as little as a thousand years
(shown here with Europa).
According to the latest theoretical models,
the gas-rich giant
planets formed first.
Jupiter probably began
as a planetary "seed" of some multiple (10 according
to some early models) of an Earth-mass located near
the planetesimal-rich snow line that then accumulated
some 300 Earth-masses of hydrogen and helium gas at
an accelerating rate while the Solar disk was still
gas rich, perhaps acquiring half its mass within only
a thousand years. It probably swept out the first
generation of asteroids by Year 2 million (where
dating of radioactive isotopes found in meteorites
indicate that the present
generation of asteroids -- which include fragments
from the iron cores of large differentiated planetesimals
and planetary embryos destroyed in massive collisions --
was formed around four million years after the birth of
the Sun). Saturn's development
may have stalled at a lower mass than Jupiter simply
because it formed a few million years later than Jupiter,
after the first gas giant to develop had already depleted
the disk's abundance of hydrogen and helium gas.
Cassini Imaging Team,
SSI,
JPL,
ESA,
NASA
Larger natural-color
image
(more).
While Jupiter helped
Saturn
to form, there was less gas
left for Saturn to bulk up with
after the first two million years
of planetary development.
The development of the gas giants from
planetary embryos was regulated by competing processes
involving the cooling of gas falling into the Sun, how
fast such infalling gas was expelled by the Sun into
interstellar space, and
type
I and II planetary migration towards the Sun.
Once a gas giant like Jupiter formed, however,
its presence probably facilitated the development of
more gas giants. It probably have flung nearby
planetesimals to farther out reaches of the Solar
System where they can form. Then Jupiter probably
helped Saturn, Uranus, and
Neptune to growth by
gravitationally pulling in gas and planetary
material that helped them form.
NASA
Larger Uranus (left) and
Neptune images.
Also known as
"ice giants,"
outer planets
Uranus and Neptune
formed later with
large rocky cores, thick icy mantles, and
thinner gas envelopes.
In the case of Uranus and Neptune, the
accumulation of planetesimals (assisted by Jupiter)
progressed farther to some 10 to 20 Earth-masses
which delayed the onset of gas accretion until too
little gas was left so that each has only around
2 Earth-masses of gas (more from
Alan
P. Boss, 2002). Uranus and Neptune are
"ice
giants," with thick icy mantles around their rocky
cores but only a relatively thin atmosphere of gas
compared with Jupiter and Saturn.
Year 10 to 100 Million - rest of
giant and
terrestrial planets develop
Although the fast formation of gas giants appears to
involve a balance of competing processes, the development
of the four rocky inner or "terrestrial"
planets (Mercury,
Venus, Earth,
and Mars) within the snow line inward
of Jupiter's orbit was slower but appears to have avoided
significant orbital migration. At the range of the terrestrial
planets' orbital distances with the snow line (0.4 to 1.5 AU
from the Sun, embryos that were composed of mostly iron and
silicate rocks grow to around 0.1 Earth-mass within 100,000
years.
After the circum-Solar disk was depleted of gas, the inner
planetary embryos gradually destabilized each other's orbits by
making them more elliptical (eccentric) over a few million years
to eventually caused more collisions. While astronomers are not
yet in agreement as to how orbital stability was subsequently
achieved among the inner planets, it is known that the four
terrestrial planets eventually merged with some of the remaining
planetesimals while deflecting the rest into the Sun over the
following 100 million years, which made their orbits more circular
and stable. As the planetary embryos grew into Mars-sized
protoplanets, however, these objects interacted gravitationally
over many orbits so that their initially circular orbits became
increasingly elliptical and they collided and merged into larger
bodies over tens of millions of years. Colliding at speeds up
to 22,000 miles per hour (36,000 kilometers per hour), such a
collision may have stripped most of the rocky mantle from the
protoplanet that became Mercury with its iron-rich core.
NASA
Larger
image.
The rocky inner planets took longer
than the giant planets to form
Dating of radioactive isotopes indicate that Mars
may have formed some 10 million years after the Sun, based on
the dating of Martian meteorites. Earth developed with most of its
final mass at around 50 million years when the proto-Earth collided
with a Mars-sized planetary embryo
("Theia")
around Year 50 million. Much of the Mars-sized embryo's core merged
with the proto-Earth's own, while the lighter ("mantle") materials
of the collision reformed as the Moon around 10 million years later
(more
discussion,
illustrations, and links and
2007
update).
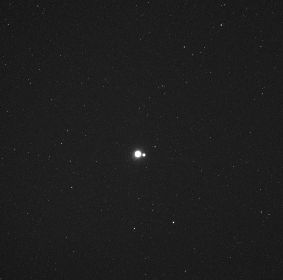
MESSENGER,
JHU-APL,
CIW,
NASA
Larger
image.
The Earth and
Moon seen
from 0.6 AUs away, around
Mercury's orbit
(more).
Year 0.1 to 1 Billion - late
planetary
migration,
gravitational
scattering, and
orbital
stability
By around Year 50 million, the dispersion of the Solar System's
larger star cluster may have perturbed some planetary orbits. As
previously discussed, some orbital instabilities may have also
developed as the Sun cleared out most of the gas within its disk. In
addition, the formation of the giant planets also led to gravitational
scattering of leftover planetesimals and planetary embryos. Uranus
and Neptune are now thought to have hurled planetesimals toward the
Edgeworth-Kuiper Belt as well as into the Sun.
Jupiter's greater gravitational might enabled it to sling planetary objects
far out into the Oort Cloud, which may contain
as much as 100 Earth-masses.
NASA --
larger
image
Further planetary migrations of the giant planets and
their gravitational scattering of leftover planetesimals
may have caused the craters of the
"late
heavy
bombardment" found on Earth's Moon.
Gravitational
scattering of planetesimals and embryos led to further
orbital migration of the remaining planets. While Neptune
and Pluto developed orbital synchrony, Saturn may have moved
closer to Jupiter before moving back outward, and caused the
so-called
"late
heavy bombardment" of the inner Solar System as dated by
craters on Earth's Moon around Year 500 to 800 million
(Bottke
and Levison, 2007; and
Gomes
et al, 2005.
Year 1 to 4.7 Billion - major post-formation cataclysms
Extended Scattered Disk objects include
2000 CR105
(which moves inward to within 44 AUs of the Sun but then outward
beyond 500 AUs) and Sedna, which never comes
closer to the Sun than 76 AUs before moving to around 900 AUs away
(into the realm of the inner Oort Cloud). Given the large orbital
eccentricities of these two objects (which move beyond 500 AUs of
the Sun), some astronomers have argued that they were likely to
have been strongly perturbed by a massive celestial object (which
is unlikely to have been Neptune as they do not come close enough
to its gravitational influence) such as a rogue planet or
passing star, which could have dragged the two objects farther
out after initial orbital perturbation by Neptune. (More
discussion on such scenarios with illustrations from computer
models are available from a
2005 Powerpoint
presentation by
Brett
Gladman and Collin Chan.)
Southwest
Research Institute
Larger illustration.
Sedna appears to move through
the outer reaches of the
Edgeworth-Kuiper Belt, which
eventually merges into the
inner Oort Cloud.
Although inclined by only around 11.9 degrees from the ecliptic
where the eight major planets orbit, Sedna's distant orbit is
extremely elliptical indicating that its formation and orbit
may have been influenced by a passing nearby star during the
early years of the Solar System, when Sol formed out of a
molecular cloud with many other closeby stars around 4.6
billion years ago. Like 2000 CR105, Sedna may have been
perturbed by a Solar-mass star at around 800 AUs from Sol more
than 100 million years after its birth, given today's observed
numbers of Oort Cloud comets
(Morbidelli
and Levison, submitted 2004).
Unlike the other planets, Uranus' axis of rotation is lies
mostly in the plane of the Solar System, as if some some titanic
collision had tipped the planet over on its side, but a new theory
suggests that its extreme tilt could have been created by a series of
smaller shifts through orbital migrations and interactions between
the giant planets during the earliest stages of Solar System formation
(Adrián
Brunini, 2006).
At a meeting of the American Astronomical
Society on October 9, 2006, two planetary scientists (Alex Alemi
and David
Stevenson) described model results which suggest that Venus may
have once had a moon that was subsequently destroyed. Under current
theories of solar system formation, Venus is unlikely to have avoided theia
a protoplanetary collision large enough to create a moon, and computer
simulations suggest that most large collisions create a debris disk
from which a moon forms. Alemi and Stevenson suggest that a sequence
of two large collisions within around 10 million years could have
first created a moon but then destroyed it and sent debris crashing
onto Venus; this eventually created the planet's slow retrograde
rotation today, which is otherwise difficult to explain
(Alemi
and Stevenson, 2006; and George Musser, Scientific American
Science
News, October 10, 2006).
Oddly enough, four of Neptune's moons are orbiting the planet within
its Roche limit. Inside that limit, Neptune's gravitation pull is so
strong that, in theory, no Solar nebular material could have
agglomerated into those satellites, and so the satellites must have
been captured or dragged within the Roche limit by tidal forces on the
planet's surface (Rist, 2000). According to
Carolyn
Porco of the University of Arizona's
Lunar and Planetary Laboratory,
these satellites may eventually disintegrate to create a ring system as
striking as those of Saturn.
Rok
Roškar (et
al, 2008)
Larger illustration.
The Sun probably has migrated far
from its birthplace due to pertubations
from the Milky Way's spiral arms
(more).
Over time, the Milky Way's spiral arms have cast the Sun far
from its birthplace, according to new numerical simulations.
As a result, stars near the Solar System vary widely in
their chemical composition because a number of them appear
to have been perturbed by the galaxy's arms into "wild" or
elongated orbits that move them far from their birthplace.
However, this perturbation by the spiral arms still preserves
the orbital circularity of many stars (including the Sun)
around the galactic center (more from
Rachel
Courtland, New Scientist, September 17, 2008;
Rok Roškar et
al, 2008; and the
Roškar
team's an animation of how the creation and
destruction of galactic spiral arms can causes stars to
migrate far from their birthplace).